Photons and electrons like to play together in atoms, but much less so in computers…
Like EUV lithography, people have been talking about optical computers for a long time, and that still hasn’t materialized.Like EUV, it seems that we’re on the verge of a major shift !
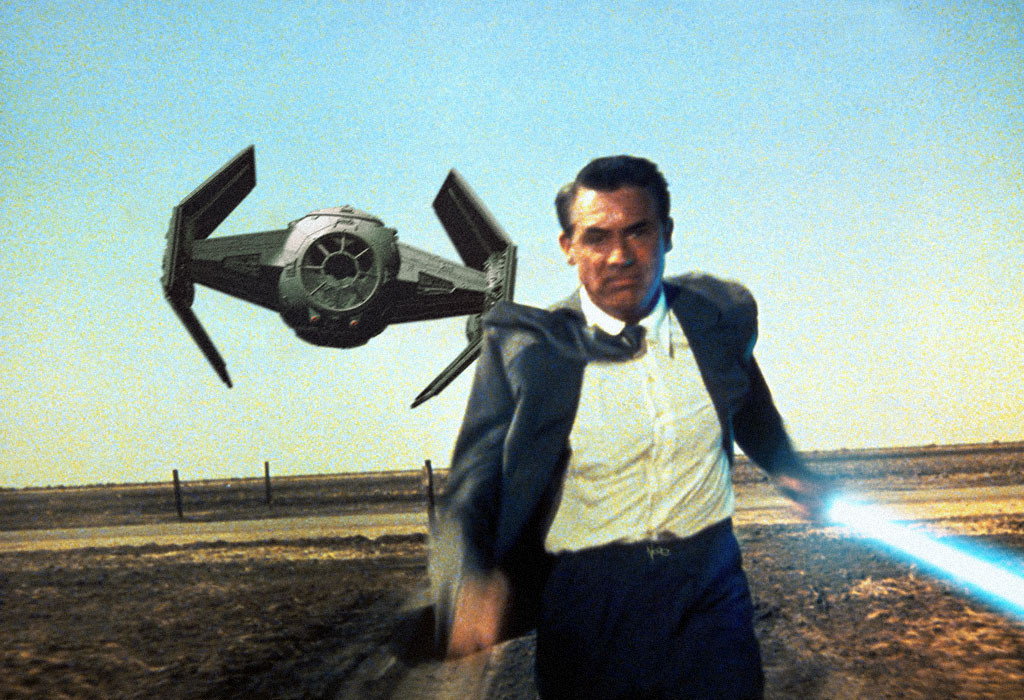
Connectivity! Cheap, fast, powerful, and energetically low-cost flows of humongous numbers of bits between chips.
Imaging a gigantic server farm with only a couple of traditional analog phone lines running into it. You have enormous power, but no way to give it the data it needs to use that power, and no way to get the result back out.Sadly, that’s pretty much the situation for most modern chips: They are IO strangled, forced to live with data rates in and out that are far less than what they could handle if given the chance. It’s like having a cheetah for a pet and keeping it in a room that only measures 5 meters by 5 meters.The reason for this lies deep into physics: We use electrons to communicate with chips, and electrons are fermions, which just means they get very ornery about being pushed too close together. So to use them, you have to create separate paths for them, and also keep them separated in time. It’s like herding cats: It only works if you define very clear paths that even an ornery cat has to stick to get anywhere.Photons in contrast are bosons, which just means they have zero problems with stepping all over each other. So for example, in a cross-connect, photons can pass right through each, and do it in huge quantities, without any problem. You can see the same effect when you cross the beams of two lasers or flashlights without any problem (well, unless you are in a Ghost Busters movie). For contrast, try crossing the fermion-based water “beams” coming out of two garden hoses and watch the splatterific results. Ditto for electrons that are not kept carefully guided at all times.Finally, photons can cross empty space, and have no annoying charges that must be cancelled out within a circuit. That makes them closest thing available to pure transfers of information.Put all of that together, and what it means is that photonic lattices can unleash the real power of all those little chips, the ones that are pacing their silicon cages in abject frustration. Everything starts to move a lot faster, and does so using far less energy.
So… photons are much better than electrons : you can pack almost as many of them as you’d like, since they interact very little with their surrounding.
But this has a serious downside : it becomes excruciatingly hard to modify the state of light (i.e. the information) that a photon carries. To do so, the most straightforward way is to use modulators. There are many kinds of them (electro-optical modulator are usual for communications, spatial light modulators more for imaging), most of them leveraging polarization effects to do so. New techniques using other exotic properties of light keep hitting the market, one of them based on the shaping of orthogonal spatial mode (my dear friend and former office mate Guillaume co-founded his company CAILabs), for increasing data rates and loss-less beam shaping of high-power lasers.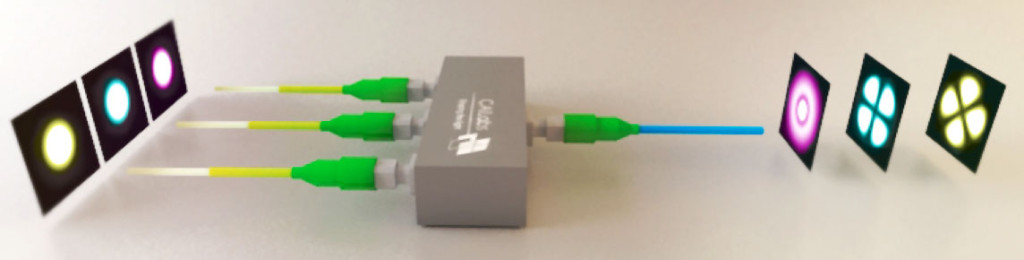
CAILcabs spatial mode multiplexer (CAILabs)
The trouble with most modulation techniques is that, since interactions occur over a long range of interaction, they usually require a minimum room space that hampers their miniaturization, and they are somehow limited in what they can do.
During my thesis, I had many times been wondering whether one could use Low-temperature grown AsGa, that has carrier lifetime in sub-picosecond range (>1THz switching capabilities, thus is why they are widely used in THz time-domain spectroscopy) to modulate the phase incurred by total internal reflection (that occurs in guided optics) and carry information… One could use femto-second lasers to do so (using the femto-second laser directly seems to be far-fetched, because of all the issues cause by dispersion). They can be cost-effective : last time I’ve inquired (4y ago), they were ~$20k and not-nearly mass produced (I believe you “just need” the right amount of dopant and be able to precisely carve chirped Bragg-mirrors) and production cost might be able to scale nicely. One day I’ll have the occasion to try that idea!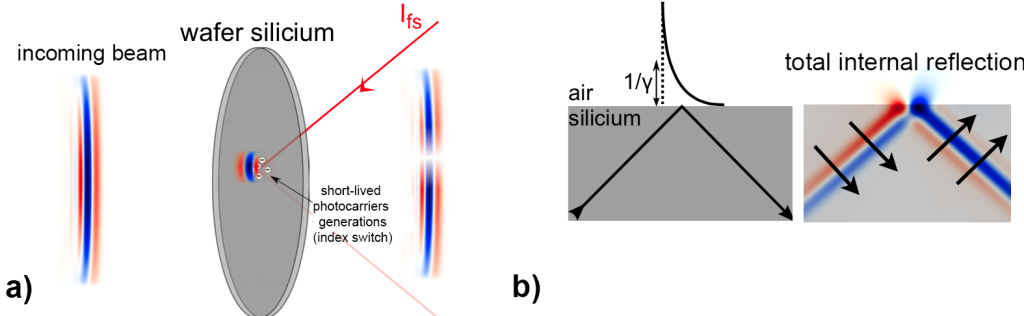
Combining two ideas for a photonics switch… (from my phd manuscript, which he the corresponding experimental data also, in French units)
Another way of carrying information is to change the way it is produced, at the source. There’s been recently different kind of approaches, and now extremely small lasers are available (see Advances in small lasers) with extremely fast switch rates (thanks to low thresholds), such as plasmon laser. In addition, controlling the source allows in certain cases to tune the frequency of the emitted light, what is probably the most difficult thing in optics, giving you an extra parameter to play with (think of how frequency modulation if fundamental in radio-communications! One can never mess with an eigen-vector of a linear-time invariant system:). Even cooler is you can operate a Single-mode laser by parity-time symmetry breaking (L. Feng et al.) !

parity-breaking optical cavity – X. Zhang’s group
Crafting the source also makes coherence effects less out-of-reach : some people playing with phase in ultrafast lasers to produce funky waveforms (see Synthesis and Measurement of Ultrafast Waveforms from Five Discrete Optical Harmonics” (Han-Sung Chan et al. – pdf)

Optical waveform synthesis using fs-lasers:) – Han-Sung Chan et al.
Another problem with optics is how to sort/route information. Since photons is so indiscriminate, cheap, compact and robust way to separate them by properties are always welcome.
A cool trick with optics is that you can often transfer some properties of your beam to an other. For example,- lens transforms positions into angles (and conversely)
- Birefringent materials transform polarization into angles
- Prisms and gratings transform wavelength to angles
The last part is probably the most important for communication/computation. Recently, approaches based on planar gratings have been proposed (Digital optical spectrometer-on-chip) sometimes using the exotic properties of random media (Compact spectrometer based on a disordered photonic chip). Halfway, one can design integrated optics couplers (Inverse design and implementation of a wavelength demultiplexing grating coupler).
Aside : it turns out that you the opposite and transform angles into wavelength (sort of : High-throughput imaging of […] objects through a single optical fibre). I don’t know if you can find a correspondence between all transformations, but figuring this could give new ideas ! Main problem with generalizing is that at some point coherence comes into play, and that’s generally a one-way street… Also, chaining this properties allows yous to do crazy stuffs, such as chirped pulse amplification, hiving birth to crazy petawatt lasers and maybe more some day, strong enough to split the vacuum!
A third problem is that you cannot store photons easily, at least not compared to electrons (storing electrons in a floating gates lets you enjoy gigabytes of music on your cell phone) : photons move at the speed of light, and when they almost always lose energy (not individually but as a whole) through absorption and reflection. To the best of my knowledge, one can only keep a photon in a confined space for a few milliseconds (see the fascination experiments of Serge Haroche, (1,2) where he uses optical cavities made of superconducting mirrors to see the birth of photons)
A Ramsey cavity to maintain photon alive for milliseconds (Sayrin et al.)
However, this three major hurdles could be circumvented if we were to do with light other things that we do with electrons. That’s the approach taken many groups, who like to use the word “quantum” in many different ways, to emphasize how cool they are and that processes are different than what is happening with electrons. They generally involves interferences (joint evolution of overlapped waves) or entanglement (joint response of photons far apart).
First, you can leverage the fact that you can superimpose tons of photons (so to speak) with different frequencies that see the world with slightly different dimensions and start Factoring numbers with interfering random waves, and break codes out-of-reach of current computers (this is actually working, though there’s a twist:)Second, you can try to minimize an objective function by using quantum tunneling between the minimas : that is called adiabatic quantum computation, and the approach championed by D-Wave. They seemed to be crackpots when I saw them giving a talk, but I would be pleased to be proved wrong…
Last, there is the Shor’s algorithm, which lying to be somewhere the two previous approaches, and which is heralded as the definitive test of quantum computation. I can’t help thinking about the day where all the data encrypted now will be transparent… Even thought they don’t commit to quantum computing yet, many companies such as HP (The Machine) or IBM (True North) are exploring new ways of computing, leveraging the capabilities of massive parallel communication.To do so, they will need to remove all the clutter created in thirty years of personal computing. If they achieve to do so, maybe there’ll be room for something else !
*
* *
It was interesting, but didn’t give much clues on the future of photonics. Principle 23 : you just can’t squeeze out any information from Intel
(they are the ones who ask me to erase data completely after they’re gone !)
[talking about DSA] On the other hand, Intel, a company that perennially listens more than it talks, is much better represented this year than in the past. – Chris Mack